Cyclicvoltammetry of O2/O2•− in aprotic solvent
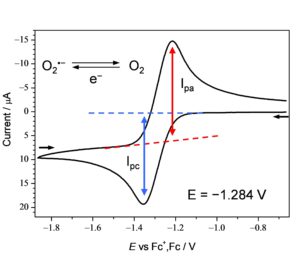
When a supporting electrolyte is added to an aprotic solvent such as acetonitrile, DMF, or DMSO to dissolve gaseous O2 and a potential is applied to the solution, O2 is reduced by one electron to generate O2•−. This situation is drawn as a current-voltage curve as shown in Figure 1 by cyclic voltammetry, which is a typical electrochemical method.
This quasi-reversible cyclic voltammogram suggests that the electrogenerated O2•− exists relatively stably and returns to O2.
O2 + e- ↔ O2•− E° = −1.284 V vs Fc+/Fc (1)
It is possible to accurately analyze the cyclicvoltammogram of O2/O2•− by the Nernst equation and digital simulation technic with measuring many conditions such as measurement temperature, electrolyte concentration, electrode area, scan rates.
Nernst equation
E = E0 + RT/zF * ln{aOx/aRed} (2)
- E0: standard redox potential
- R: gas constants
- T: temperature (K)
- z: number of electron
- a: activity coeficient
- F: Faraday constants (96 485 C mol−1)
Electrogenerated superoxide radical anion: O2•−
In aprotic solvents, O2•– is electrogenerated via one-electron reduction of O2 at −1.284 V versus the ferrocenium ion/ferrocene (Fc+/Fc) couple (eq 2). The reactivity between electrogenerated O2•– and added substrates is observable in modification of reversible CVs of O2/O2•– (Fig.1).
In the presence of acidic substrate such as phenols, the loss of reversibility in the O2/O2•– is due to the proton transfer (PT) from the phenols forming hydroperoxi radical (HO2•, eq 3) and subsequent reactions (eq 4-5).
O2•− + PhOH → HO2• + PhO− proton transfer (3)
HO2• + e- → HO2− E° = −0.4 to −0.2 V vs Fc+/Fc (4)
HO2•+ PhO− → HO2− + PhO• electron transfer (5)
Reactivity of electrogenerated O2•−
In the electrochemical study of O2, O2•− is electrogenerated and the reactivity of O2•− toward sample is observed by coexisting in the solution. O2•− becomes a doublet radical by adding an electron to the π-orbital of the triplet oxygen molecule (Figure 1 red arrow), and is metabolized while becoming a precursor of the subsequent ROA in the reaction process.
By adding biological components such as cells, DNA, proteins, and various pharmaceuticals to O2•− generated by the reduction of O2, it is possible to analyze its oxidation mechanism by O2•−.
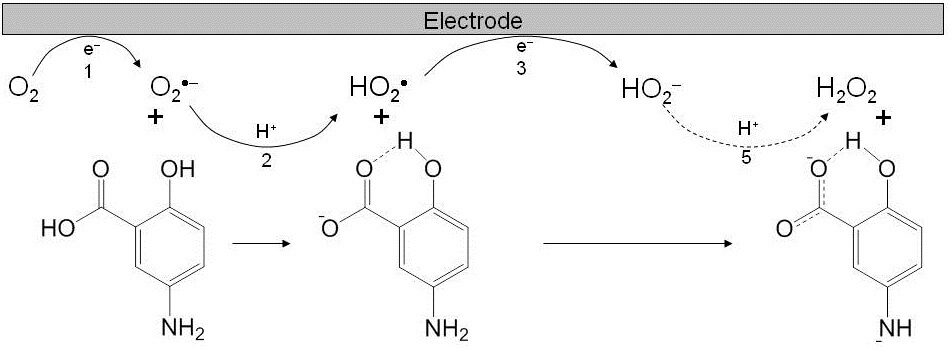
Related papers
- Importance of Proton-Coupled Electron Transfer from Natural Phenolic Compounds in Superoxide Scavenging
- Chem. Pharm. Bull. 2015, 63, 12, 967-973
- https://doi.org/10.1248/cpb.c15-00447
- Structural Properties of 4-Substituted Phenols Capable of Proton-Coupled Electron Transfer to Superoxide
- Int. J. Adv. Res. Chem. Sci. 2016, 3, 1, 11-19
- http://dx.doi.org/10.20431/2349-0403.0301002
- Quinone–Hydroquinone π-Conjugated Redox Reaction Involving Proton-coupled Electron Transfer Plays an Important Role in Scavenging Superoxide by Polyphenolic Antioxidants
- Chem. Lett. 2010, 39, 3, 162-164
- https://doi.org/10.1246/cl.2010.162
- Superoxide and Hydroperoxyl Radical Spin Trapping Using 5,5- Dimethyl-1-Pyrroline N-Oxide in Dehydrated N,N-Dimethylformamide
- tatsushi nakayama
- Preprint https://papers.ssrn.com/sol3/papers.cfm?abstract_id=3707605